-
oa “Nanomaterials and Environmental Biotechnology”
Edited by Indu Bhushan (Shri Mata Vaishno Devi University, India), Vivek Kumar Singh (Shri Mata Vaishno Devi University, India), Durgesh Kumar Tripathi (Amity University, India), Nanotechnology in the Life Sciences Series, Springer Nature Switzerland AG, Cham, Switzerland, 2020, 434 pp, ISBN: 978-3-030-34543-3, £129.99, €155.99, US$179.99
- Source: Johnson Matthey Technology Review, Volume 64, Issue 4, Oct 2020, p. 526 - 528
-
- 01 Jan 2020
Preview this article:
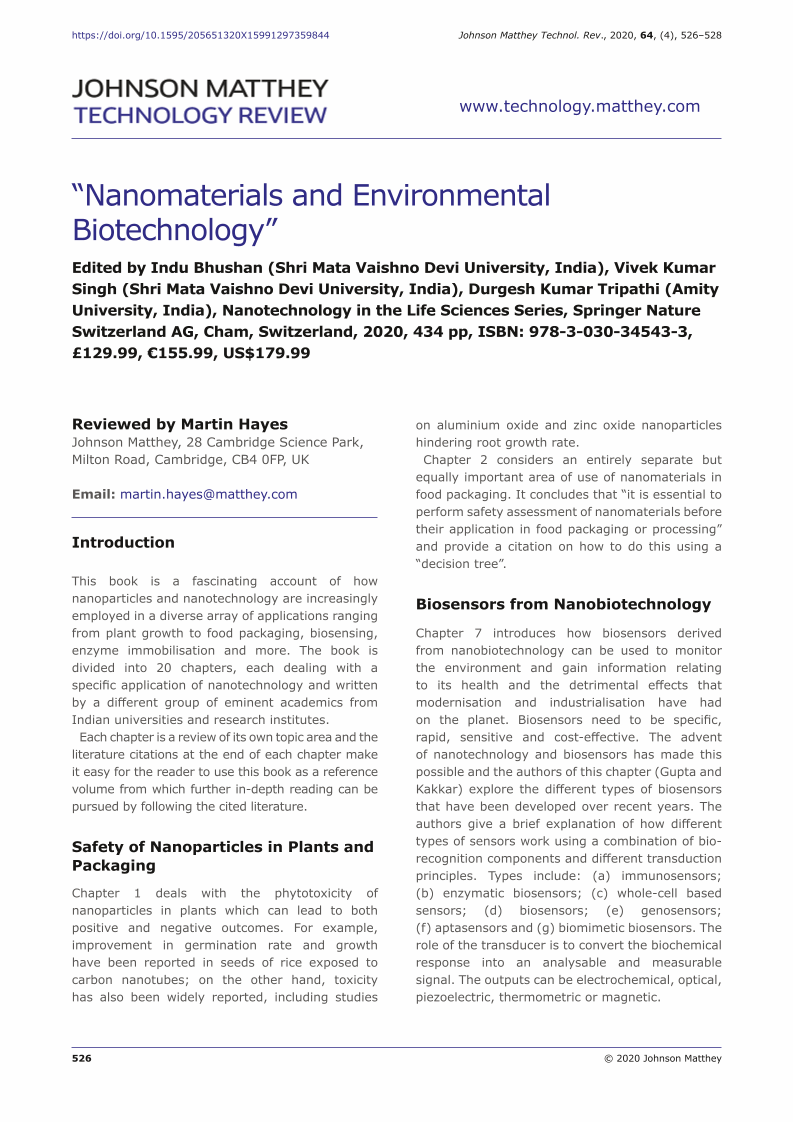



“Nanomaterials and Environmental Biotechnology”, Page 1 of 1
< Previous page Next page > /docserver/preview/fulltext/jmtr/64/4/Hayes_16a_Imp-1.gif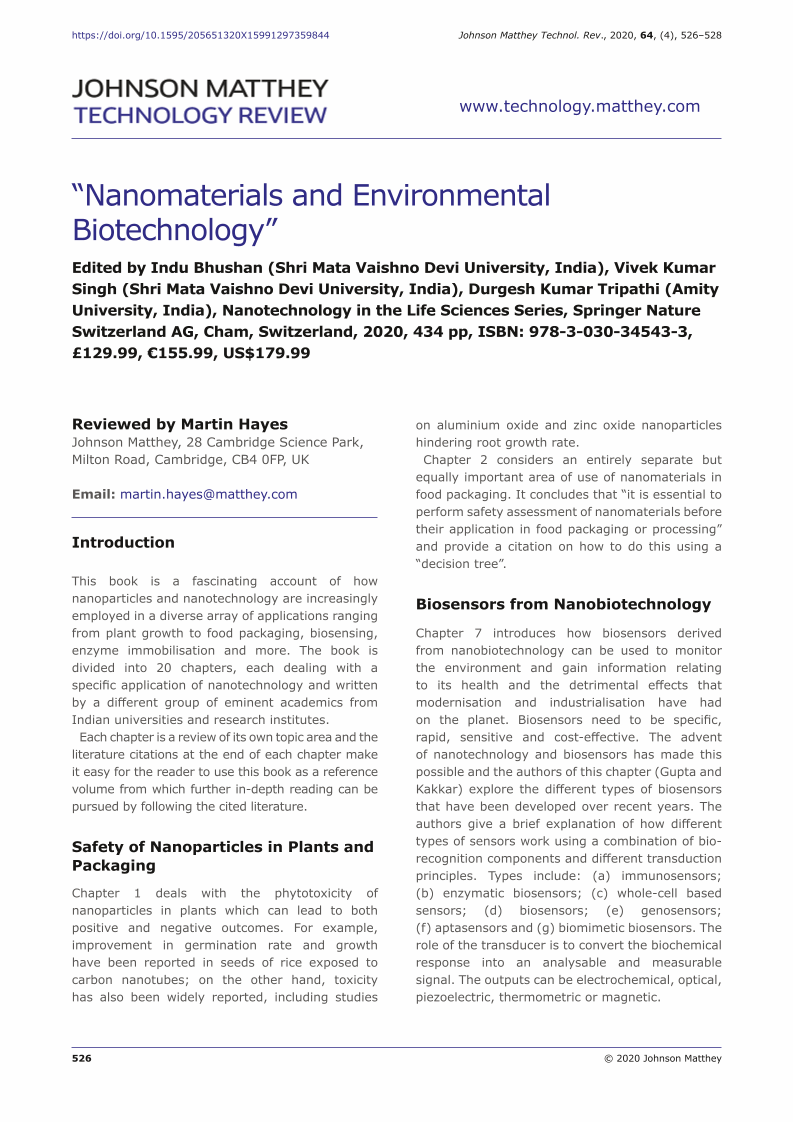
There is no abstract available.
© Johnson Matthey