-
oa Guest Editorial: Biocatalysis and Pharmaceuticals
- Source: Johnson Matthey Technology Review, Volume 60, Issue 4, Oct 2016, p. 226 - 227
-
- 01 Jan 2016
- Previous Article
- Table of Contents
- Next Article
Preview this article:
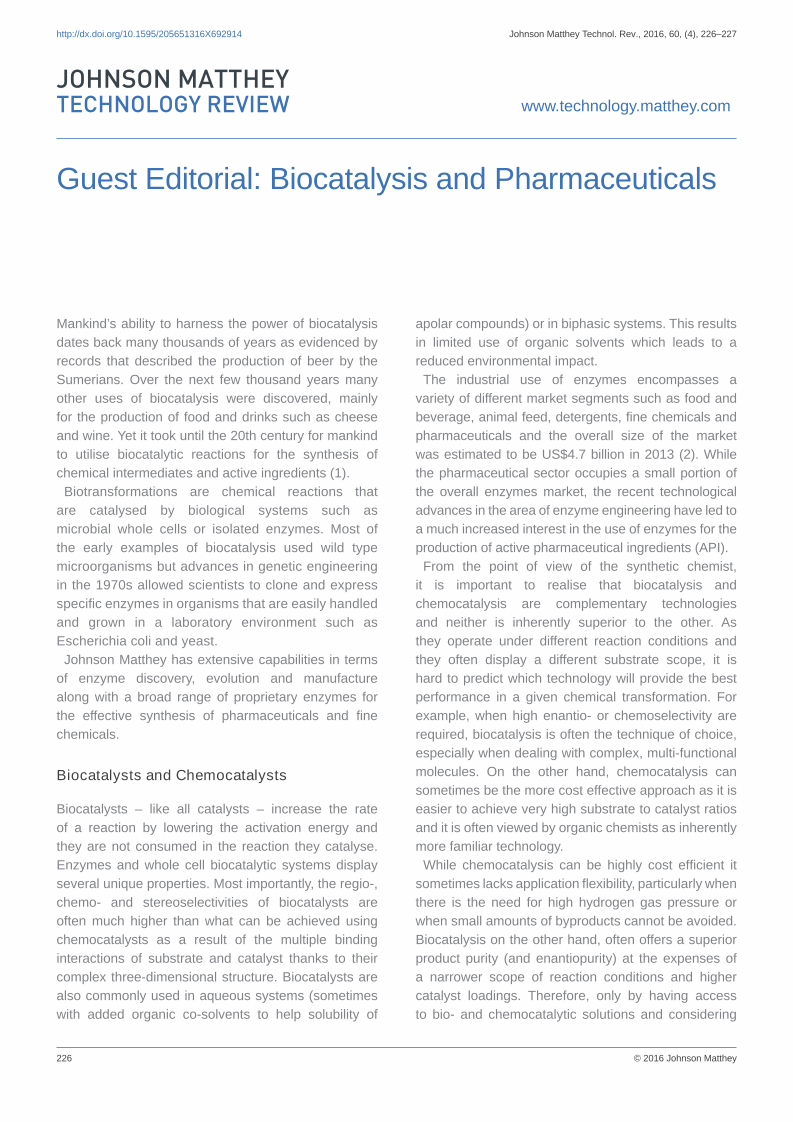



Guest Editorial: Biocatalysis and Pharmaceuticals, Page 1 of 1
< Previous page Next page > /docserver/preview/fulltext/jmtr/60/4/JMTR-60-4-Biocatalysis_Editorial-1.gif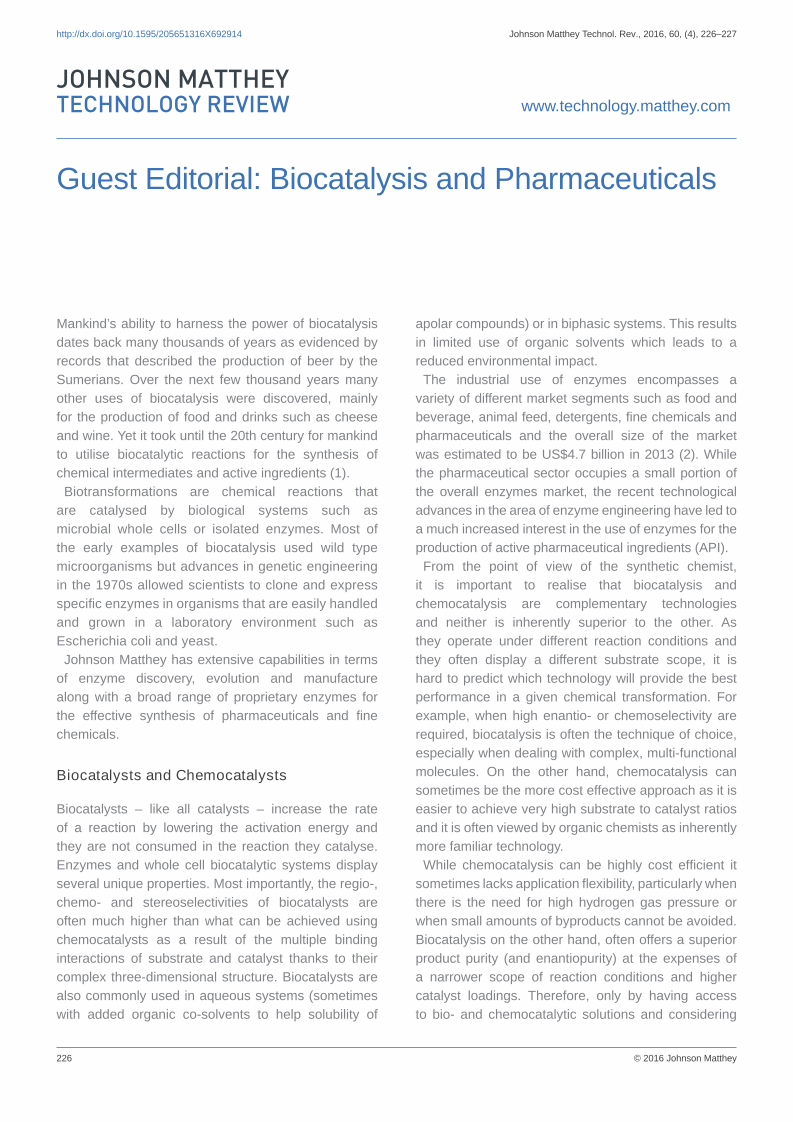
There is no abstract available.
© Johnson Matthey