-
oa Lithium Sulfur Batteries: Mechanisms, Modelling and Materials Conference
Recent advances in lithium-sulfur batteries research
- Source: Johnson Matthey Technology Review, Volume 61, Issue 4, Oct 2017, p. 308 - 310
-
- 01 Jan 2017
Preview this article:
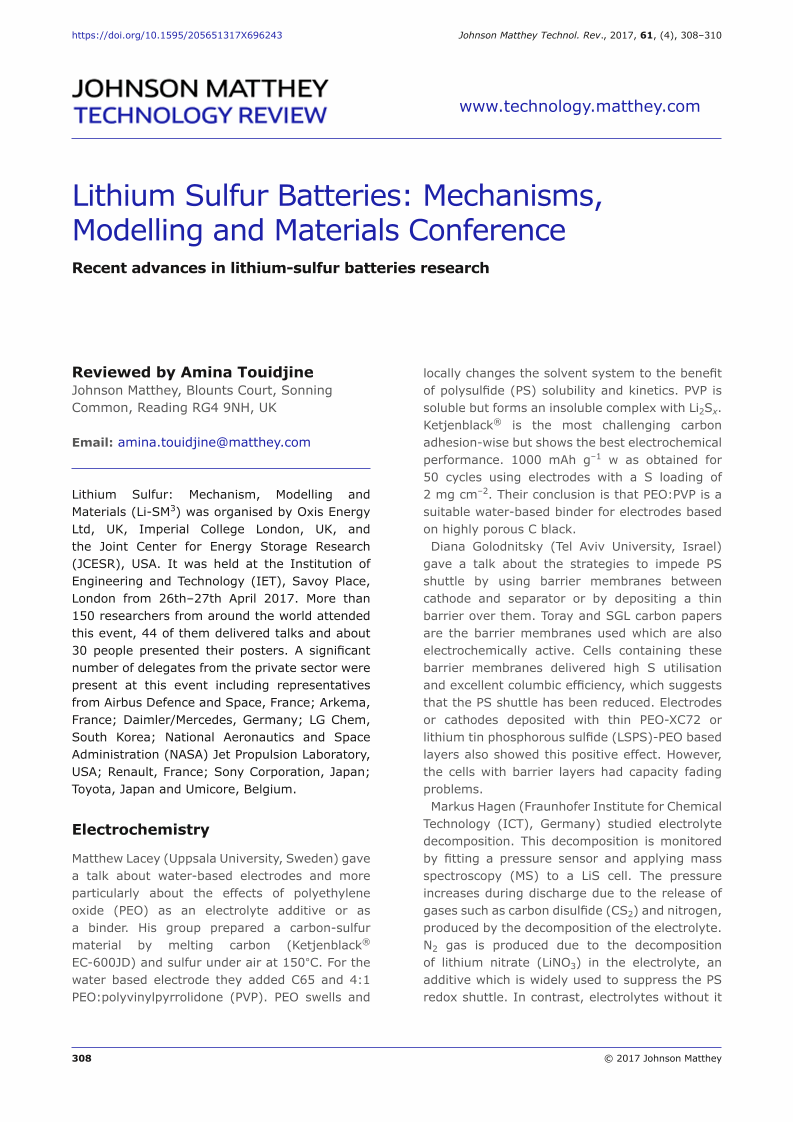



Lithium Sulfur Batteries: Mechanisms, Modelling and Materials Conference, Page 1 of 1
< Previous page Next page > /docserver/preview/fulltext/jmtr/61/4/Touidjine_16a_Imp-1.gif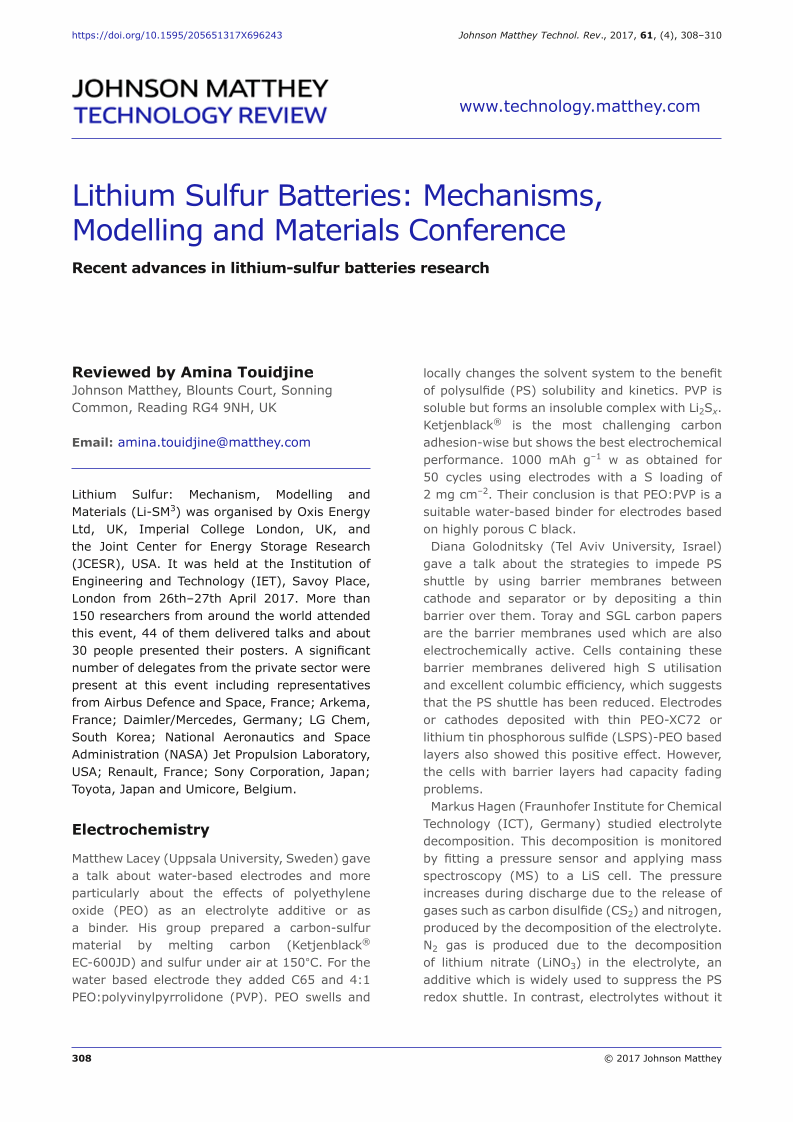
There is no abstract available.
© Johnson Matthey