-
oa In the Lab: Spotlight on Surface Characterisation Activities at Johnson Matthey
Johnson Matthey Technology Review features laboratory research
- Source: Johnson Matthey Technology Review, Volume 66, Issue 1, Jan 2022, p. 77 - 80
-
- 23 Nov 2021
- 05 Jan 2022
Preview this article:
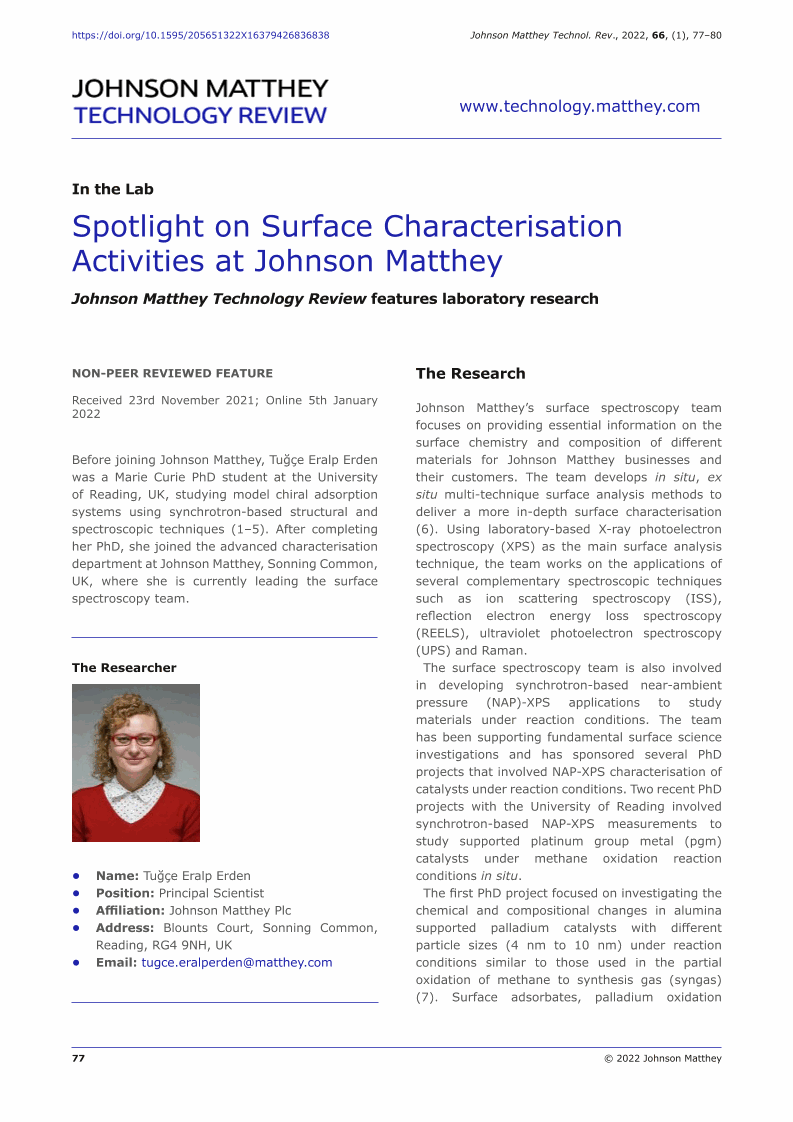



In the Lab: Spotlight on Surface Characterisation Activities at Johnson Matthey, Page 1 of 1
< Previous page Next page > /docserver/preview/fulltext/jmtr/66/1/Erden_16a_Imp-1.gif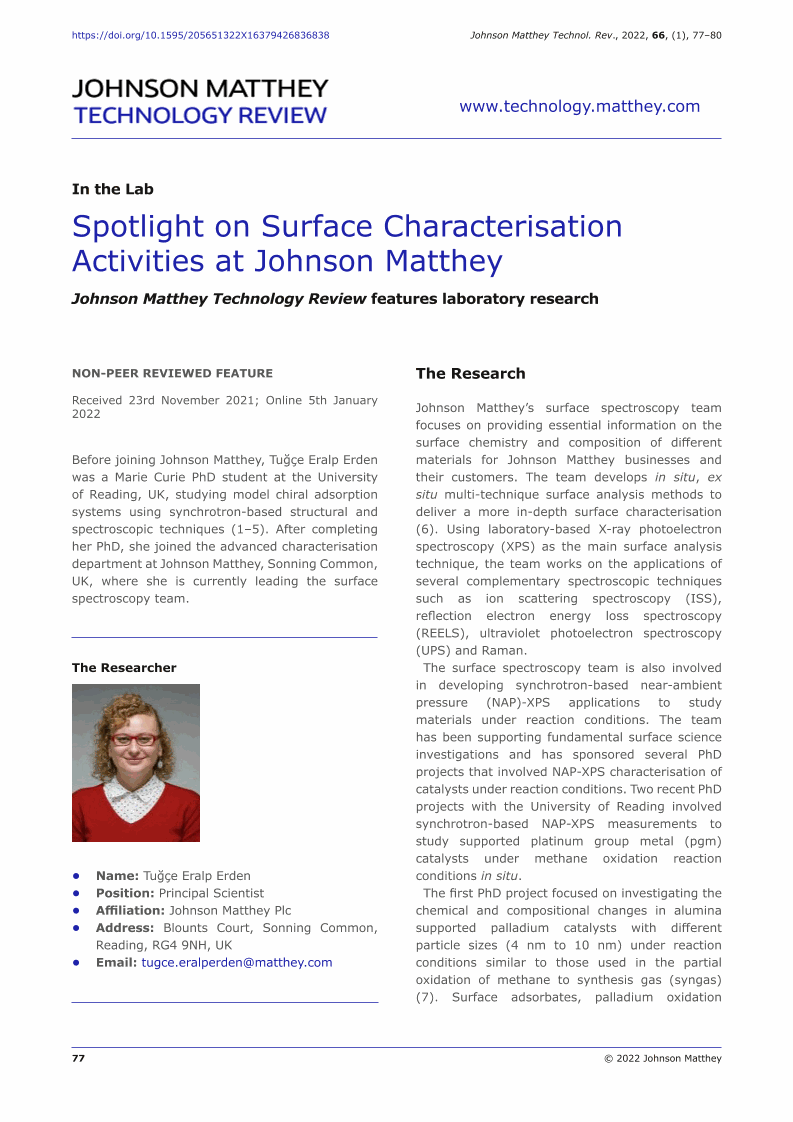
There is no abstract available.
© Johnson Matthey